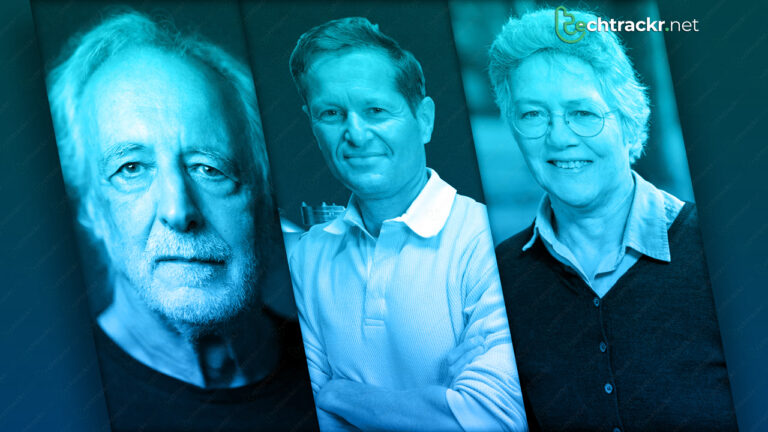
If you want to see those super speedy particles in the tiny world of subatomic stuff, you gotta create super quick bursts of light. Anne L’Huillier, Pierre Agostini, and Ferenc Krausz just won the 2023 Nobel Prize in Physics for their groundbreaking work in making it possible to shed light on things happening in unbelievably short moments.
From the ’80s to the early 2000s, these three scientists came up with ways to create laser bursts that only last attoseconds – a time frame way shorter than a blink of an eye. When you look at things in these tiny, quick bursts, the world seems to take its sweet time. The superfast flutter of a hummingbird’s wings feels like it lasts forever, and even the constant chatter of atoms seems to move in slow motion. On the attosecond scale, scientists can actually see electrons moving around atoms, hopping from one spot to another.
A new era of attochemistry
Aside from being a totally fresh way of studying electrons, this method of slowing down our view of the world could open up loads of practical uses. According to Mats Larsson, a member of the Nobel committee, it kickstarted the whole field of “attochemistry,” where you can tinker with individual electrons using light.
Send attosecond laser bursts at a semiconductor, and it goes from blocking electricity flow to conducting it in a flash. This could lead to super speedy electronic devices. Plus, one of this year’s Nobel Prize winners, Krausz, is looking to use attosecond pulses to spot tiny changes in blood cells that might hint at the early stages of cancer.
The ultrafast world is like a whole different realm, but thanks to the work of L’Huillier, Agostini, Krausz, and their fellow scientists, we’re starting to get a glimpse of it.
Also read: How an Omega watch changed the way NASA fixes the ISS
Here’s what an attosecond means
Just to put it in perspective, an attosecond is a tiny sliver of time, like 0.000000000000000001 seconds. There are more attoseconds in a single second than there have been seconds since the universe was born.
When it comes to tracking planetary orbits, we use days, months, and years. For something quick like a 100-meter sprint, we turn to seconds or even hundredths of a second. But when we venture into the ultra-tiny world of atoms and particles, things happen at lightning speed. To catch the almost-instant dances of electrons, we need super-precise stopwatches with incredibly tiny tick marks – attoseconds.
Back in 1925, Werner Heisenberg, a quantum mechanics trailblazer, said that we can’t really observe the time it takes for an electron to make a lap around a hydrogen atom. In a way, he was spot on. Electrons don’t do this neat little orbit like planets around a star. Instead, physicists view them as a kind of probability wave, showing where and when we might spot them. So, we can’t measure an electron in the traditional sense of it zipping through space.
However, in a different way, Heisenberg didn’t quite anticipate the cleverness of 20th-century physicists like L’Huillier, Agostini, and Krausz. Those electron odds of being in one place or another are constantly changing, from one tiny attosecond to the next. With the power to generate these ultra-quick attosecond laser bursts that can interact with electrons in real-time, scientists can directly investigate different electron behaviors.
How are attosecond pulses produced?
Back in the ’80s, Ahmed Zewail over at the California Institute of Technology figured out how to make lasers flicker with super short pulses that lasted just a few femtoseconds – which are thousands of attoseconds. These quick flashes, for which Zewail scored the 1999 Nobel Prize in Chemistry, were a game-changer, letting scientists delve into the intricate dance of chemical reactions between atoms in molecules. It was dubbed “the world’s fastest camera.”
For a while, getting an even faster camera seemed like a tough nut to crack. Nobody was sure how to push light to oscillate any faster. However, in 1987, Anne L’Huillier and her team stumbled upon something intriguing: When they shone light on specific gases, the atoms got all jazzed up and started spitting out additional colors of light that oscillated way faster than the original laser – a phenomenon called “overtones.” The curious part was that in gases like argon, some of these extra colors were brighter than others, but they followed an unexpected pattern. At first, physicists were scratching their heads trying to make sense of it.
In the years that followed, physicists put this newfound knowledge of overtones to work and started creating attosecond pulses in the lab. Agostini and his team came up with a technique they called “Rabbit,” short for “reconstruction of attosecond beating by interference of two-photon transitions.” Using Rabbit in 2001, Agostini’s gang managed to generate a series of laser pulses, each as short as 250 attoseconds. In the same year, Krausz’s crew used a slightly different approach called “streaking” to whip up and study individual bursts, each hanging around for 650 attoseconds. However, L’Huillier and her colleagues outdid them all in 2003, producing a laser pulse that lasted just 170 attoseconds.
Also read: What’s the minimum number of people required for a sustainable Mars habitat?
What are the applications of these attosecond pulses?
With attosecond pulses, scientists can observe anything that changes within a range of dozens to hundreds of attoseconds. Their initial goal was to tackle what physicists had deemed nearly impossible (or at the very least, highly unlikely): gaining a clear view of the precise actions of electrons.
Way back in 1905, Albert Einstein fired up the whole quantum mechanics thing when he explained the photoelectric effect. This is when you shine light on a piece of metal and it shoots out electrons (he later scored a Nobel Prize in 1921 for this idea). Before the era of attosecond physics, scientists typically thought that the sequence of reactions causing those electrons to be set free happened instantly.
In 2010, Krausz and his team proved this assumption wrong. They employed attosecond pulses to time electrons getting booted from neon atoms. What they discovered was that an electron in a lower-energy state made a run for it 21 attoseconds quicker than one in a higher-energy state. Fast forward to 2020, another group of scientists demonstrated that electrons make a quicker getaway from liquid water compared to water vapor, with a time difference measured in tens of attoseconds.
Researchers are actively working on exploring more uses for attosecond pulses. This innovative technique could dig into a range of electron activities, such as how they transport and obstruct electric charge, interact with each other, and exhibit collective behaviors. Krausz is even using attosecond bursts to study human blood. Just last year, he played a role in revealing that tiny alterations in a blood sample can signal the presence of early-stage cancer and help identify the specific type.